Ca2+ channel nanodomains boost local Ca2+ amplitude.
Date
2013-09-24
Journal Title
Journal ISSN
Volume Title
Repository Usage Stats
views
downloads
Citation Stats
Attention Stats
Abstract
Local Ca(2+) signals through voltage-gated Ca(2+) channels (CaVs) drive synaptic transmission, neural plasticity, and cardiac contraction. Despite the importance of these events, the fundamental relationship between flux through a single CaV channel and the Ca(2+) signaling concentration within nanometers of its pore has resisted empirical determination, owing to limitations in the spatial resolution and specificity of fluorescence-based Ca(2+) measurements. Here, we exploited Ca(2+)-dependent inactivation of CaV channels as a nanometer-range Ca(2+) indicator specific to active channels. We observed an unexpected and dramatic boost in nanodomain Ca(2+) amplitude, ten-fold higher than predicted on theoretical grounds. Our results uncover a striking feature of CaV nanodomains, as diffusion-restricted environments that amplify small Ca(2+) fluxes into enormous local Ca(2+) concentrations. This Ca(2+) tuning by the physical composition of the nanodomain may represent an energy-efficient means of local amplification that maximizes information signaling capacity, while minimizing global Ca(2+) load.
Type
Department
Description
Provenance
Subjects
Citation
Permalink
Published Version (Please cite this version)
Publication Info
Tadross, Michael R, Richard W Tsien and David T Yue (2013). Ca2+ channel nanodomains boost local Ca2+ amplitude. Proc Natl Acad Sci U S A, 110(39). pp. 15794–15799. 10.1073/pnas.1313898110 Retrieved from https://hdl.handle.net/10161/15559.
This is constructed from limited available data and may be imprecise. To cite this article, please review & use the official citation provided by the journal.
Collections
Scholars@Duke
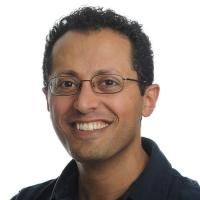
Michael Raphael Tadross
Dr. Tadross' lab develops technologies to rapidly deliver drugs to genetically defined subsets of cells in the brain. By using these reagents in mouse models of neuropsychiatric disease, his group is mapping how specific receptors on defined cells and synapses in the brain give rise to diverse neural computations and behaviors. The approach leverages drugs currently in use to treat human neuropsychiatric disease, facilitating clinically relevant interpretation of the mapping effort.
He received his B.S. degree in Electrical & Computer Engineering at Rutgers University, an M.D.-Ph.D. degree in Biomedical Engineering at the Johns Hopkins School of Medicine, and completed his postdoctoral study in Cellular Neuroscience at Stanford University. He began his independent research program as a fellow at the HHMI Janelia Research Campus.
Unless otherwise indicated, scholarly articles published by Duke faculty members are made available here with a CC-BY-NC (Creative Commons Attribution Non-Commercial) license, as enabled by the Duke Open Access Policy. If you wish to use the materials in ways not already permitted under CC-BY-NC, please consult the copyright owner. Other materials are made available here through the author’s grant of a non-exclusive license to make their work openly accessible.