Effect of surface topography on in vitro osteoblast function and mechanical performance of 3D printed titanium.
Date
2021-10
Journal Title
Journal ISSN
Volume Title
Repository Usage Stats
views
downloads
Citation Stats
Abstract
Critical-sized defects remain a significant challenge in orthopaedics. 3D printed scaffolds are a promising treatment but are still limited due to inconsistent osseous integration. The goal of the study is to understand how changing the surface roughness of 3D printed titanium either by surface treatment or artificially printing rough topography impacts the mechanical and biological properties of 3D printed titanium. Titanium tensile samples and discs were printed via laser powder bed fusion. Roughness was manipulated by post-processing printed samples or by directly printing rough features. Experimental groups in order of increasing surface roughness were Polished, Blasted, As Built, Sprouts, and Rough Sprouts. Tensile behavior of samples showed reduced strength with increasing surface roughness. MC3T3 pre-osteoblasts were seeded on discs and analyzed for cellular proliferation, differentiation, and matrix deposition at 0, 2, and 4 weeks. Printing roughness diminished mechanical properties such as tensile strength and ductility without clear benefit to cell growth. Roughness features were printed on mesoscale, unlike samples in literature in which roughness on microscale demonstrated an increase in cell activity. The data suggest that printing artificial roughness on titanium scaffold is not an effective strategy to promote osseous integration.
Type
Department
Description
Provenance
Subjects
Citation
Permalink
Published Version (Please cite this version)
Publication Info
Abar, Bijan, Cambre Kelly, Anh Pham, Nicholas Allen, Helena Barber, Alexander Kelly, Anthony J Mirando, Matthew J Hilton, et al. (2021). Effect of surface topography on in vitro osteoblast function and mechanical performance of 3D printed titanium. Journal of biomedical materials research. Part A, 109(10). pp. 1792–1802. 10.1002/jbm.a.37172 Retrieved from https://hdl.handle.net/10161/25648.
This is constructed from limited available data and may be imprecise. To cite this article, please review & use the official citation provided by the journal.
Collections
Scholars@Duke

Nicholas Allen
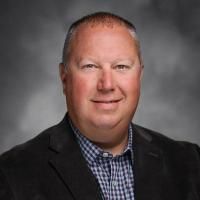
Matthew James Hilton
A long-term interest of the Hilton lab is to uncover the molecular circuitry regulating lineage commitment, proliferation, and differentiation of skeletal stem cells, chondrocytes, and osteoblasts. My laboratory uses genetic mouse models and primary cell culture techniques coupled with biochemistry to answer questions regarding skeletal stem cell self-renewal/differentiation, chondrogenesis, and osteoblastogenesis. Recently my lab has generated novel data from a variety of Notch gain and loss-of-function mutant mice demonstrating the importance of Notch signaling in each of these processes. We are currently investigating the exact Notch signaling mechanisms at play during skeletal development, disease, and repair. Additional studies are also focused on identifying and understanding the molecular mechanisms underlying various congenital skeletal pathologies, including Multiple Herediatry Exostoses (MHE) and Preaxial Polydactyly (PPD).

Ken Gall
Professor Gall’s research aims to develop a fundamental understanding of the relationship between the processing, structure, and mechanical properties of materials. His scientific contributions range from the creation and understanding of shape memory metals and polymers to the discovery of a new phase transformation in metal nanowires. His current research interests are 3D printed metals and polymers, soft synthetic biomaterials, and biopolymers with structured surface porous networks.
In addition to his research he has consulted for industry, the US Military and the US Intelligence Community, and served as an expert witness in multiple patent and product litigations. Finally, he is a passionate entrepreneur who uses fundamental scientific knowledge to hasten the commercialization of new materials and improve the effectiveness of existing materials. He founded two medical device start-up companies, MedShape and Vertera who have commercialized university based technologies in the orthopedic medical device space.
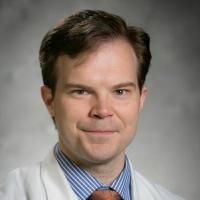
Samuel Bruce Adams
Unless otherwise indicated, scholarly articles published by Duke faculty members are made available here with a CC-BY-NC (Creative Commons Attribution Non-Commercial) license, as enabled by the Duke Open Access Policy. If you wish to use the materials in ways not already permitted under CC-BY-NC, please consult the copyright owner. Other materials are made available here through the author’s grant of a non-exclusive license to make their work openly accessible.